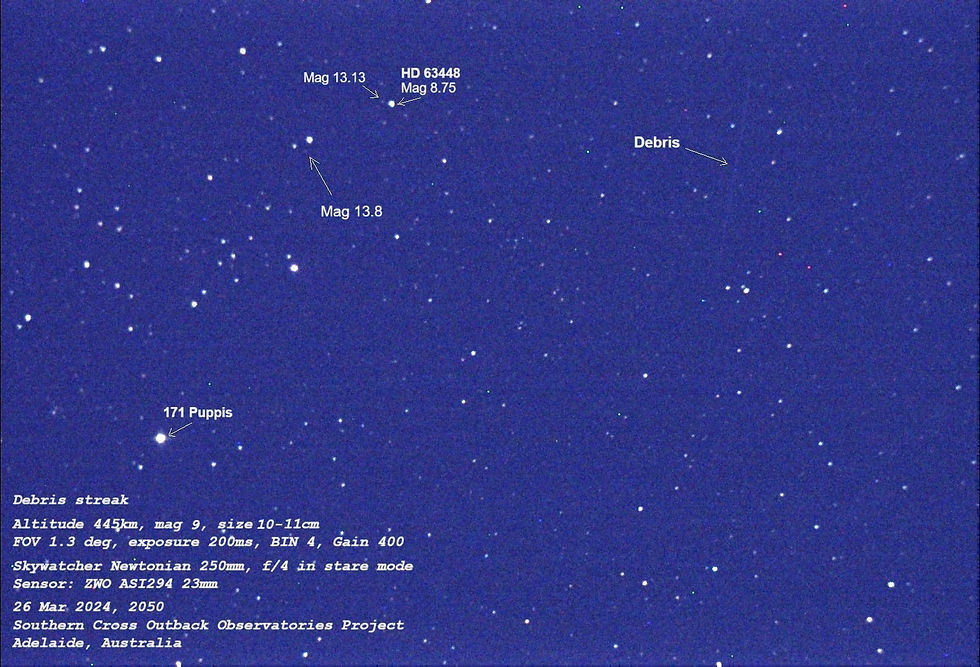
Our patent-pending Trace, Track and Tackle sequence to detect and mitigate space debris starts with the primary detection of uncatalogued debris. 1-10 cm uncatalogued debris in the LEO (Low Earth Orbit) is our area of primary interest, not only because it is undetected and untrackable, but it is still large enough to be lethal for satellites and human space missions. Any bigger (>10cm), and it is fewer in number and mostly catalogued. Any smaller (<1cm), though far more numerous and considered harmful, it is not likely to be lethal for space missions. It is the 1-10cm debris which is bad news.
It is known by different names; Dr Darren McKnight of LeoLabs calls it Lethal Non-Trackable Debris. We call it the Dark Debris. One scathing peer review of our TTT concept even called this class of debris as “it simply does not exist”, implying it is a waste of time and resources to try developing and implementing any techniques to detect and counter this class of debris.
This makes it our area of interest to waste some time and resources…our favorite pastime!
Since the debris we are looking for is within a size-range which is mostly undetected and uncatalogued through optical means we must devise special techniques to detect it in a way that can create some sort of basic trajectory parameters. Since this debris is uncatalogued, it means there is no data available to aim and track the telescope to detect or study it. This also means there is no point in using a tracking mount. Just simply stare at a fixed spot and hoping to catch a streak.
In order to develop a basic trajectory of an RSO (Resident Space Object), be it a satellite or a piece of space junk, we need to capture a streak that falls within the margins of the frame. The streak length in arc-seconds can be calculated by knowing the FOV (field of view) of the telescope and the sensor being used, and is usually the fraction or percentage of the diagonal of the frame. The streak length will help us estimate the altitude of the RSO which has a set velocity at a given altitude. The higher the RSO is, the shorter the streak length in arc-seconds, not only for its distance but also for its slower orbital speed. Online calculators can be used to find the velocity of the RSO for a particular altitude such as the Omni Calculator.
As the RSO is moving fast, it will form an end-to-end streak on the screen on longer exposure times, and won’t help estimation of the speed and altitude of the RSO. Long exposure also does not add to the brightness of the streak in the long exposure frame. A shorter exposure, in range of 100-400ms will be sufficient to capture a streak in full length.
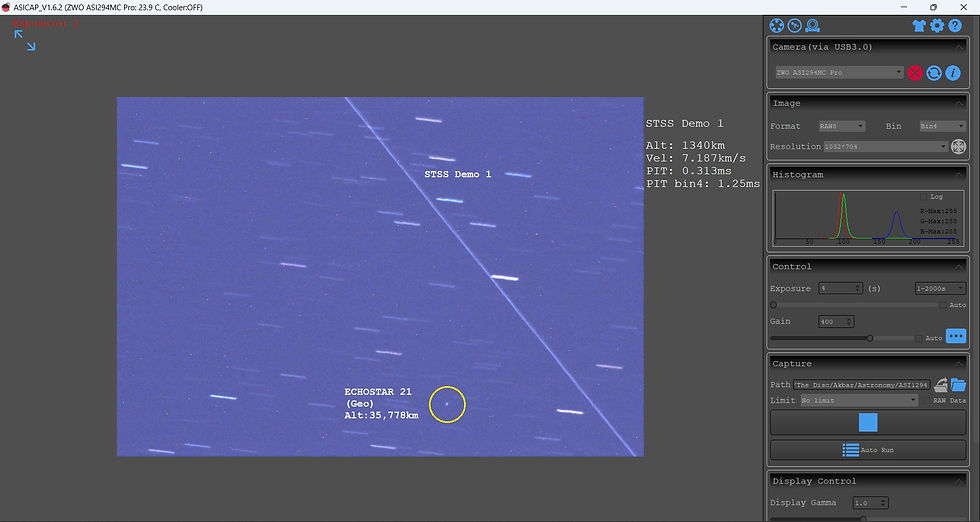
This is a 4s exposure of a region near Betelgeuse on 11 Mar 2023. A LEO satellite created an entire streak due to long exposure.

This is Starlink satellite captured in stare mode transiting the M42 Orion Nebula on 17th Mar 2023. Reducing the exposure to 200ms creates a streak within the frame. The length of the streak can help calculate the altitude with a fair amount of accuracy. Here the calculated altitude simply from the streak is 560km, against the catalog altitude of 546km at that moment in time.
These observations were done using a very large focal ratio telescope, the Celestron C11 with a focal length of 2800mm and a focal ratio of f/10. The disadvantage of such a long focal length is the narrow field of view and thus a very limited pixel integration time (PIT) or the time light from the object stays on a single pixel.
During these observation runs, we only captured live and defunct satellite streaks and those of spent last stages or rocket bodies, but none in the range of size of a cubesat or a 1-10cm debris. In order to detect objects in this size and magnitude range, we needed to increase the PIT through the use of a telescope of similar aperture but smaller focal length (focal ratio). This meant larger FOV and slower transit of light from an orbital object over the sensor, hence increased PIT and increased chances of its detection.
Skywatcher 250mm f/4 Quattro with a focal length of 1000mm was a decent choice of equipment for this purpose.
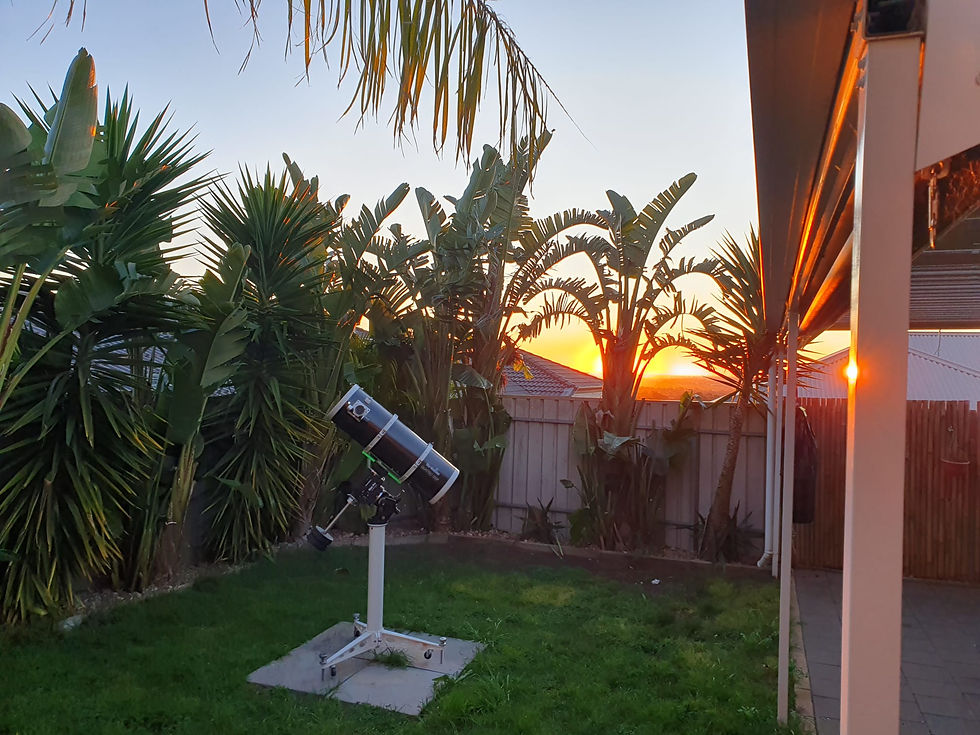
(Skywatcher 250mm f/4 Quattro on Orion Atlas Pro AZ/EQ-G mount)
In August 2023, we had tested it in stare-mode (on a powered-off mount), i.e. gazing at a fixed spot at the sky. We had captured plenty of streaks of known and unknown RSOs including active and defunct satellites and rocket bodies. As the 250 Quattro covered nearly 1.3 degrees FOV on the 23mm ASI294 sensor, the streaks were shorter and brighter for the same exposure as compared to Celestron C11 with a FOV of 0.46 degrees on the same sensor. This was due to increased PIT on the larger FOV by the Quattro.
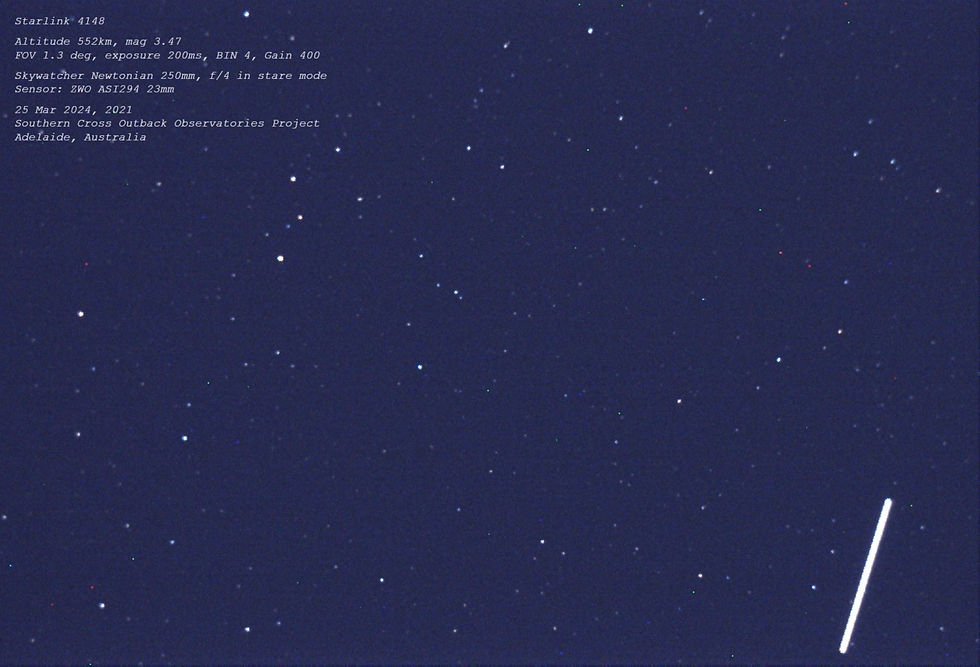
(Starlink satellite from Skywatcher 250 f/4 Quattro. Note the shorter and much brighter streak for the same exposure as compared to the Starlink streak from Celestron C11 f/10 (above) for nearly the same altitude. This is due to the longer PIT for the Quattro, resulting in brighter streak)
As we conducted the study in stare mode, the mount was unnecessary. We considered putting the Quattro OTA on a firm surface such as a wooden footstool like a bucket, and point it directly upwards to stare at Zenith.
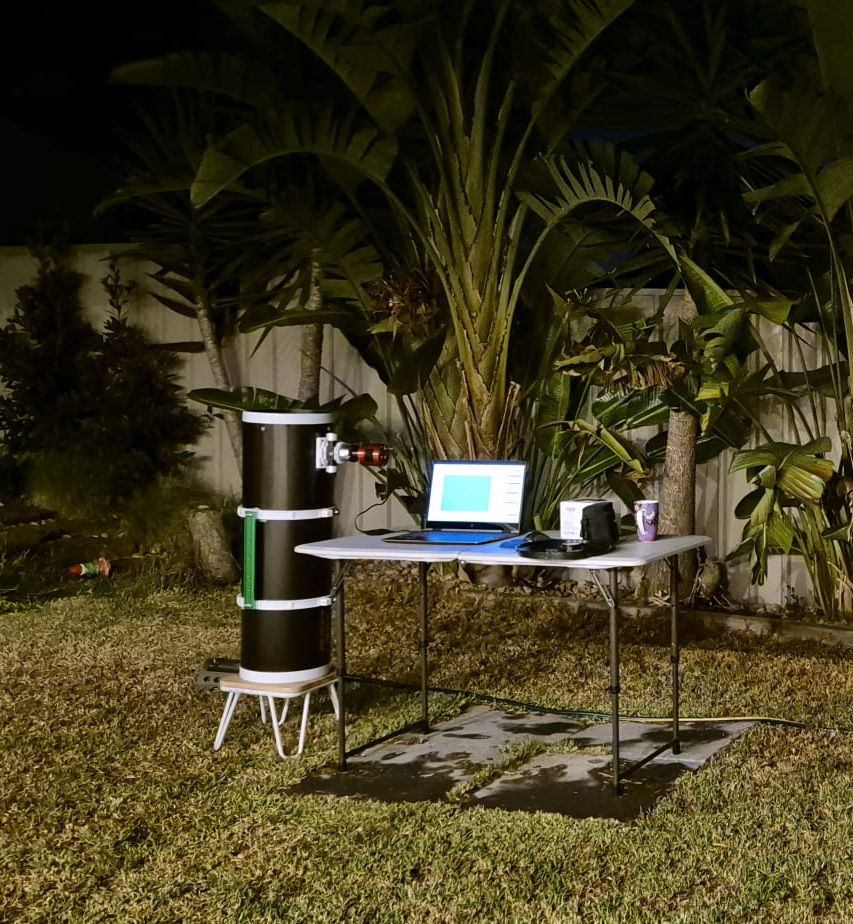
We conducted a test run on 20th March 2023. Among several streaks from larger active and dead satellites and rocket bodies, we detected a faint reproducible streak, almost to the extinction limit.

Upon further enhancement,
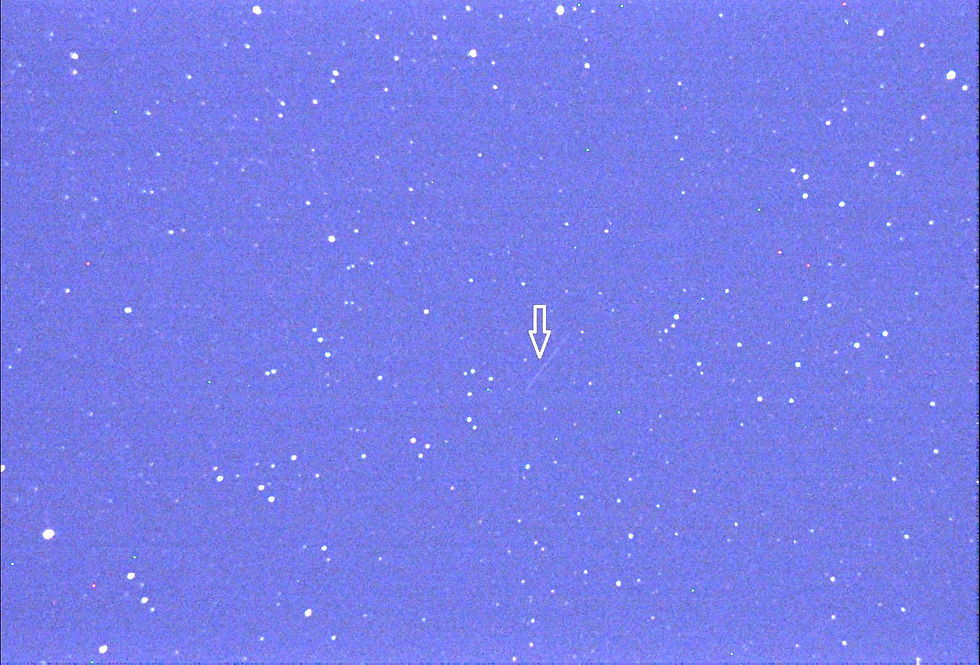

Using Omni Calculator, its altitude comes as nearly 1000km, with an orbital speed of 7.345km/s
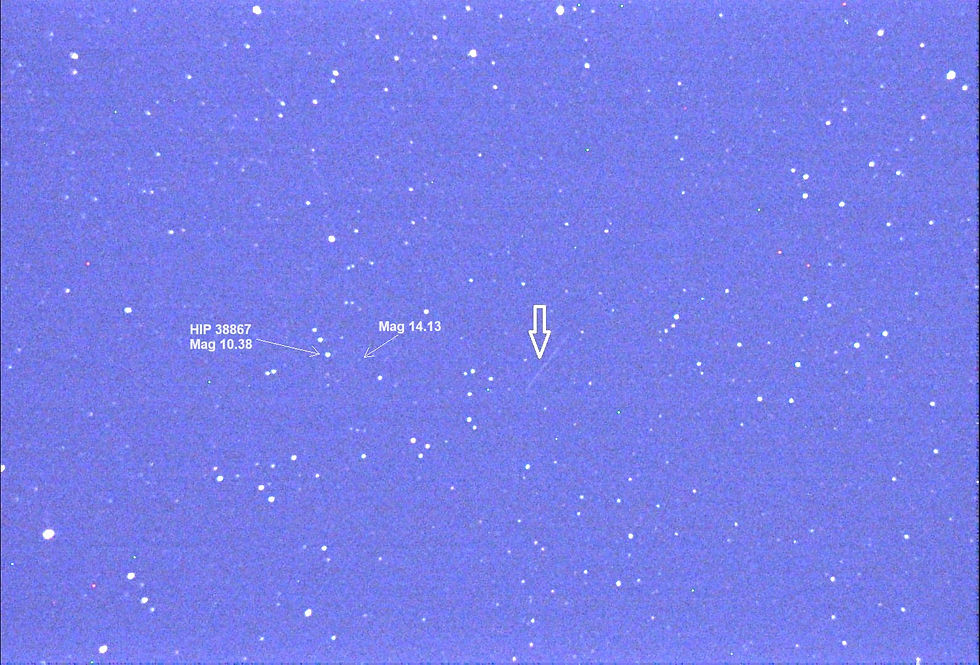
The faintest known star in this image is a magnitude 14.13 star (labelled). We used it to calculate the magnitude of the object forming the streak. Though the streak seems much brighter, a single fragment down to the pixel level is quite faint, something like one order of magnitude than the 14.13 magnitude star. But this is enough to calculate the magnitude of the streak-forming object. This can be calculated as follows.
Calculations
The diagonal of the frame covers 1.3 degrees which spans 5013 pixels (Sensor dimensions of 4144 x 2822).
No of pixels per mm of the diagonal of the sensor = 5013/23 = 218pixels/mm
Dimensions of 1 pixel = 1/218 = 0.0046mm, or 1/5013 x1.3 = 0.00026 degrees
The streak length is 6.45% of the diagonal (20mm vs 310mm on the computer screen) which comes as 323 pixels.
Exposure: 200ms
Angle subtended by the streak = 6.45/100 x 1.3 = 0.0838 degrees in 200ms
Sidereal rate of stars = 360 degrees /86400s = 0.00416 degrees/s
In 200ms: 0.00416/5 = 0.000833 degrees
Number of pixels covered by stars in 200ms = 0.000833/0.00026 = 3.2 pixels
Since it is a stare mode, the stars are also making a slow streak over the exposure period spanning 3.2 pixels in 200ms.
Ratio of debris streak vs stellar streak in 200ms = 323:3.2 = 323/3.2 = 100.9 ~ 100
This means the debris streak would be a 100 times brighter spot if it was moving at a sidereal rate on the sensor.
Magnitude difference: A 100 times brightness corresponds to 5 magnitude difference.
As the debris streak is likely to be 1 magnitude fainter than the faintest star in the frame (Mag 14.13), the magnitude of the debris is nearly 15.
Subtracting 5 from it gives us a magnitude of 10
With two known parameters i.e altitude of 1000km and magnitude of 10, the size of the debris can be estimated from the following chart
(Blake J.A., Optical imaging of space debris in low and high altitude orbits, University of Warwick)
According to the chart of debris magnitude with size and altitude, we can determine the size of this debris for its brightness and approximate altitude.
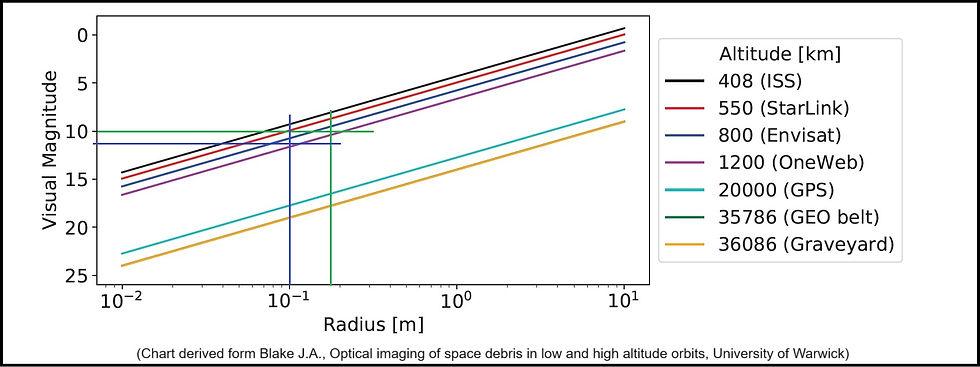
This chart shows that a magnitude 10 object at 1000km altitude will be nearly 11cm in size. This chart also shows that an 10cm object at that altitude will have a magnitude of 11.
The magnitude of a piece of debris depends upon several factors such as shape, colour and material of the object, so these figures are approximations within a reasonable limit.
Putting the calculated values on this chart of the visual magnitudes of the debris for their size and altitudes, this streak turns out to be from a LEO debris of 8-9cm in size at nearly 1000 km altitude.
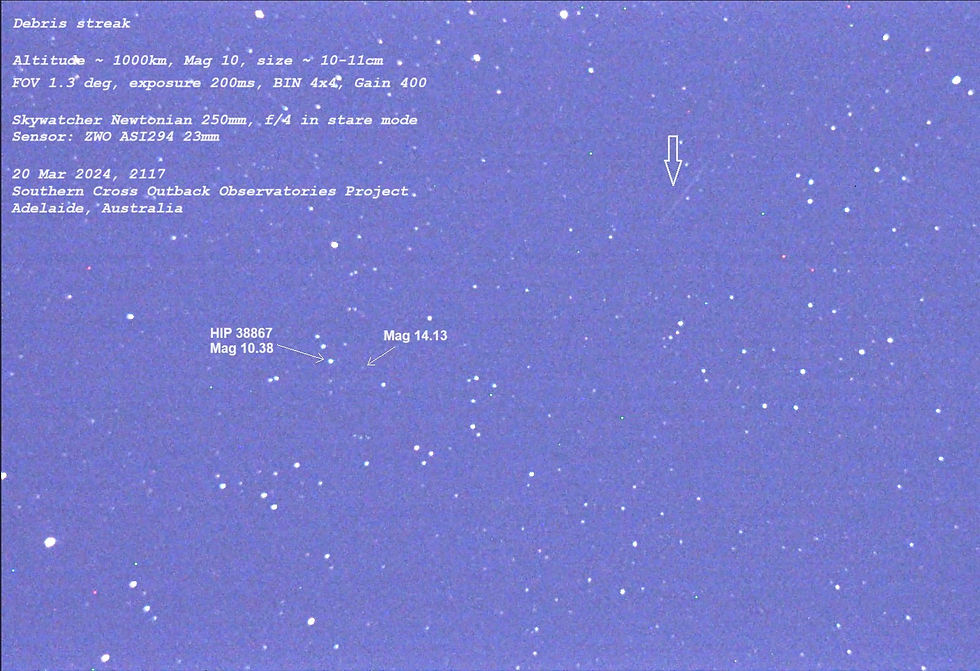
So our inference is that this is indeed a LEO debris of around 10cm size which we were able to not only detect, but were also able to determine the basic orbital and trajectory parameters of, based on its length and direction, and position in the sky.
How faint is this streak, here is an example of a known satellite body for comparison, the Intelsat-22 rocket body at 3300km altitude captured during the same study run at exactly 2121 Adelaide Time. Our calculations based on the length of its streak shows its altitude of 3400-3500km. Even this object is extremely faint at a magnitude of 7.23. An object of magnitude 11.6 will be 54 times fainter, which is not unthinkable provided the faintness of our observed 8-9cm streak as compared to the Intelesat-22 rocket body streak.
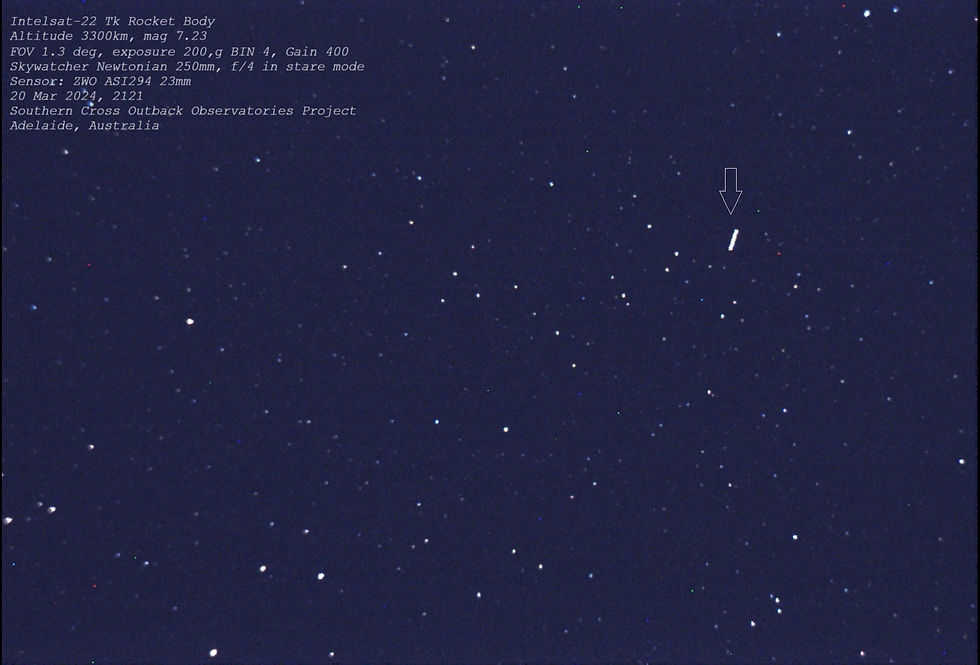
Lets analyse another streak from our test run on 26th Mar 2024.
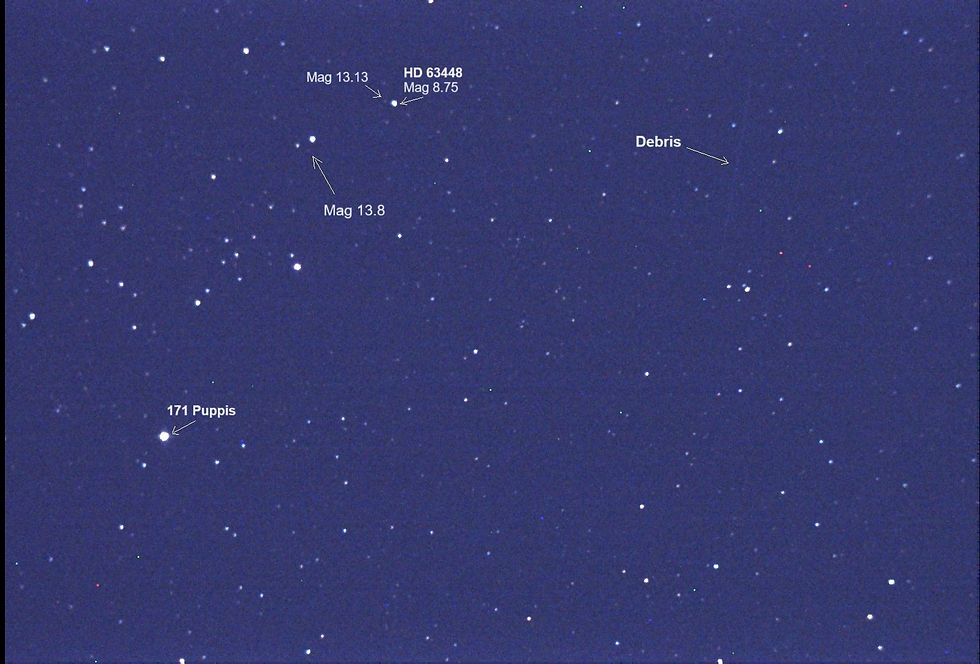
Calculations
The faintest known star in this image is mag 13.8
The streak length here is 15.16% of the diagonal (47mm vs 310mm on the computer screen) which comes as 760 pixels.
Exposure: 200ms
Angle subtended by the streak = 15.16/100 x 1.3 = 0.197 degrees in 200ms
In 1 sec: 0.985 degree
Using Omni Calculator, its altitude comes as nearly 445km, with an orbital speed of 7.647km/s
Ratio of debris streak vs stellar streak in 200ms = 760:3.2 = 760/3.2 = 238 ~ 240
This means the debris streak would be a 240 times brighter spot if it was moving at a sidereal rate on the sensor.
Magnitude difference: A 240 times brightness corresponds to 5.98 magnitude difference or roughly 6.
As the debris streak is likely to be 1 magnitude fainter than the faintest star in the frame (Mag 13.8), the magnitude of the debris is nearly 14.8.
Subtracting 6 from it gives us a magnitude of 8.8 ~ 9
With two known parameters i.e altitude of 445 km and magnitude of 9, the size of the debris can be estimated from the following chart

This chart shows that a magnitude 9 object at 445km altitude will be nearly 10-11cm in size.
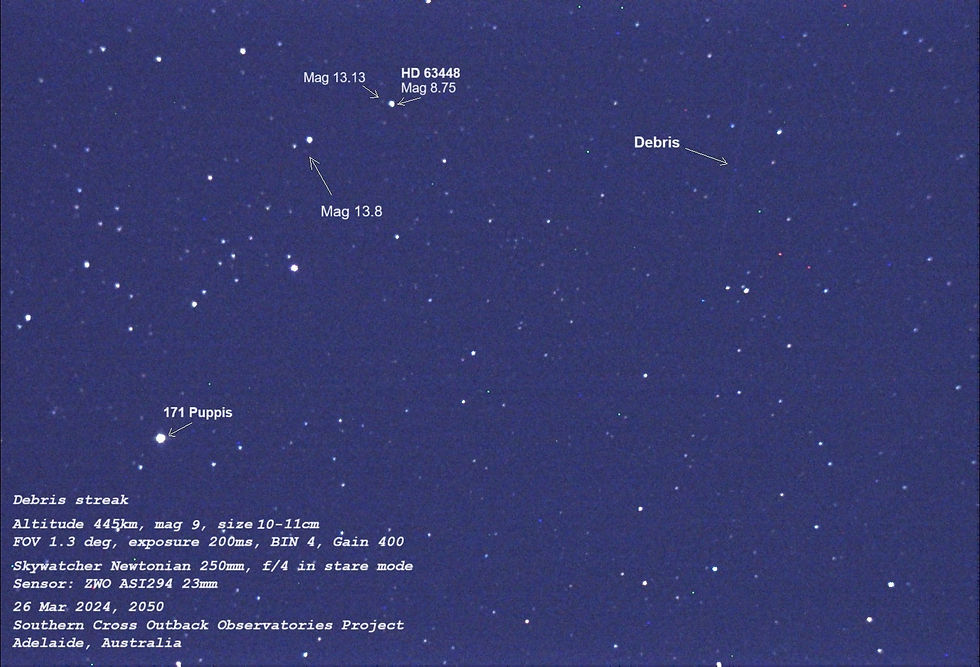
This completes our initial assessment of the possibility of detecting a 10cm object in Low Earth Orbit using the detection component of our patent-pending Trace, Track and Tackle sequence, and it gave us affirmative results.
Conclusion
If by using an average amateur astronomy gear, one can not only detect but also estimate its basic parameters means 1-10cm LEO debris is not as hard a game as conventionally believed. Working with better telescopes and sensors and operating in the darkest and clearest skies of the Australian outback, one may crank up the detectability to 1cm debris at 2000km, which is the lowest limit of our aims and objectives of detecting and mitigating the LEO debris through our patent-pending trace, track and tackle sequence.
コメント